What Are Macros? Breaking Down The Big Three Macronutrients
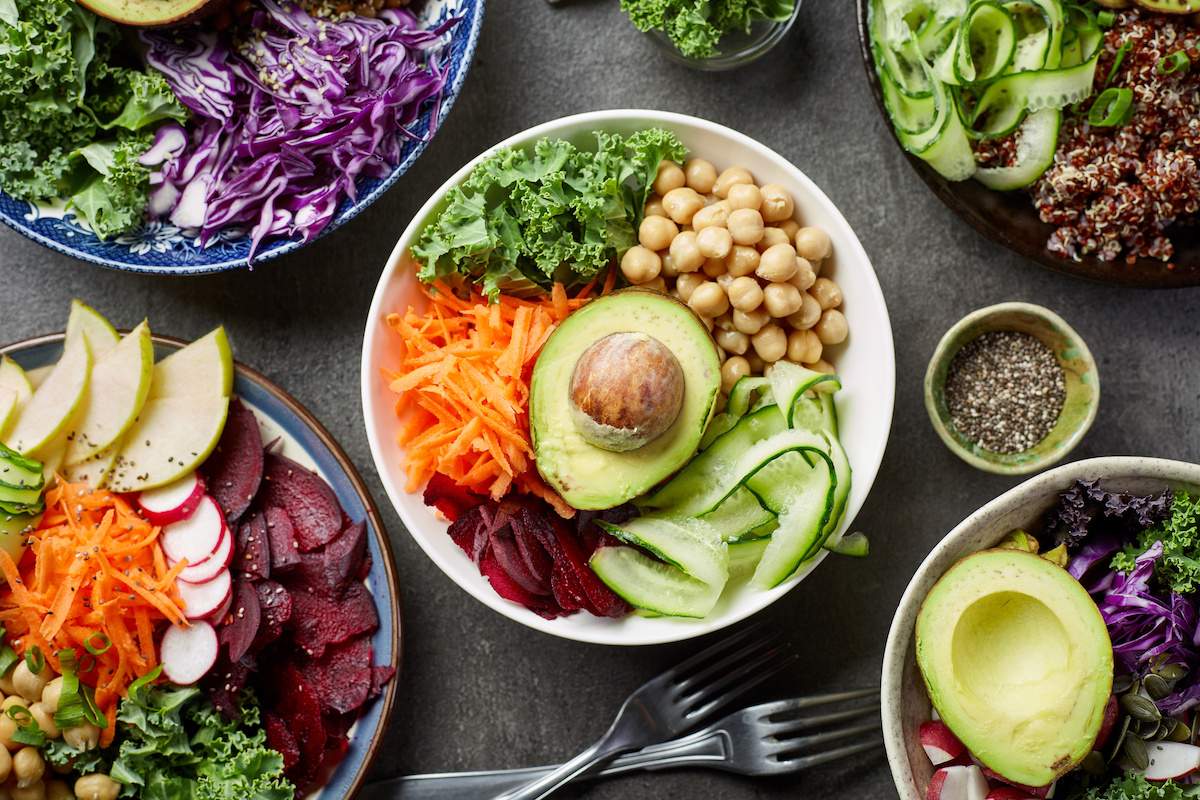
Nutrients are best defined as specific substances in food and drink that are required for normal body function and need to be supplied by the diet. Surprisingly, despite a huge range of substances found in what we eat, especially in a westernised diet, the list of true nutrients is relatively finite. These true nutrients are categorised based on how much you need to consume (e.g. daily), splitting nutrients into macronutrients, or macros, and micronutrients.
Macronutrients, as the name suggests, are required in relatively large amounts, i.e. grams per day, whereas micronutrients are required in much smaller amounts, milligrams (a 1000th of a gram), or even just micrograms (a millionth of a gram) per day.
Macronutrients are required in large regular amounts because they are either used as fuel or provide building materials for making key body molecules and the growth and repair of body tissues. There are only three macros: carbohydrate, fat (or more accurately lipid) and protein.
However, there is one other non-nutrient component of the diet that also provides energy (i.e. serves as a fuel) which isn’t an actual macronutrient but should be considered alongside them, and that is alcohol. Micronutrients, in contrast, are not actually needed in large regular amounts because although they are integral to metabolic function and normal physiology, they are not themselves “consumables” as such. These are your vitamins and minerals.
Individual dietary requirements for macronutrients (and micronutrients) are fundamentally based on metabolic demand. The ability to meet this metabolic demand will in turn be a consequence of how much of these nutrients you have to start with, as well as other factors such as the ability to absorb the nutrients from the diet, ability to store and utilise the nutrient, higher turnover or loss, or the presence of disease or infection. These in turn are also influenced by somatic factors such as age, ethnicity and gender.
Nevertheless, understanding what these nutrients are and their role is required before we can begin to understand metabolism and its application.
The First Macro: Carbohydrates
Many explorations of the macronutrients often begin with carbohydrates, which have been somewhat demonised of late but are arguably the most fundamental macronutrient when it comes to metabolism. So to begin with we need to be clear what we mean by carbohydrates?
Monosaccharides
Well, when categorising carbohydrates by molecule complexity, the simplest forms of carbohydrates are monosaccharides (“one sugar unit”), with the three main monosaccharides being glucose, galactose and fructose.
From a metabolic perspective, we tend to focus almost exclusively on glucose, as this is the form of carbohydrate we circulate and utilise for energy and storage in the body. However, we very rarely consume these on their own in our diets, as most of the carbohydrate in food and drink exists in several other forms.
Disaccharides
Disaccharides (“two sugar units”), are the next form of carbohydrate in terms of complexity and represent specific pairings of monosaccharides commonly found in the diet. The most well-known of these is sucrose — containing both glucose and fructose — which makes up what we typically think of as sugar. Both monosaccharides and disaccharides are collectively coined “simple sugars” and are mainly found in fruits (e.g. fructose), milk and other dairy products (e.g. lactose), or more commonly added to foods specifically (e.g. sucrose). Simple sugars are generally digested and absorbed rapidly due to their uncomplicated structure.
Complex Carbohydrates
For many people, the bulk of carbohydrates consumed in the diet are in the form of “complex carbohydrates”. As the name suggests, these are more complex carbohydrate molecules, and mainly relate to polysaccharides (many sugar units), which are large carbohydrate molecules made up of 10 or more monosaccharides joined together. For many, the most common polysaccharide in the diet (and indeed complex carbohydrate generally) is starch, which is made up exclusively glucose molecules.
Despite their complexity, we are well equipped to digest and absorb food starch polysaccharides like potatoes and rice, especially after traditional cooking, and these can provide a rapid delivery of carbohydrate into the system.
Maltodextrins
Modern diets have also begun to use maltodextrins which are man-made polysaccharides derived from starch that are relatively short, typically up to 20 glucose units in length. These are used as food additives and are often found in soft drinks and sports products. They are very rapidly digested and absorbed, often quicker than some simple sugars, with shorter length maltodextrins most quickly absorbed and sweeter in taste.
The Liver As A Gatekeeper
All these forms of carbohydrate can be digested and absorbed by the human gastrointestinal system or the “gut” for final breakdown into individual monosaccharides (glucose, fructose and galactose). All absorbed monosaccharides go to the liver first, before any chance of reaching the wider, systemic circulation that supplies everything else. In short, the liver is a key gatekeeper in the supply of glucose as a substrate to all other tissues.
Don’t Forget About Fibre
So far, we have described those forms of carbohydrate that are digested and absorbed efficiently in the human gut. But the term “complex carbohydrates” covers a whole array of other forms of carbohydrate which are not digested in the traditional sense. These collectively represent what we call fibre. A big proportion of fibre comprises of non-starch polysaccharides (NSPs), with cellulose the main example which can be found in plant cell walls.
We can’t directly harness the energy in fibre components ourselves, nevertheless, all components of fibre can provide good fodder for the resident bacteria in our gut which can break these down by fermentation. The breakdown products of this fermentation can actually be useful to us (e.g. short-chain fatty acids serving as a fuel for the cells of the gut wall themselves) and can have significant metabolic influence beyond energy or fuel provision.
The Second Macro: Fat
Fat, or more collectively lipids, represent a family of molecules that chemically are water-insoluble, yet have a variety of key metabolic functions. Under the umbrella of lipids we include the energy sources of triacylglycerides (TAG) or fatty acids, but also cholesterol and other structural lipids, as well as functional lipids as we shall explore in this section.
Fatty Acids
The basic units of lipid, from which all lipids originate, are fatty acids, chains of hydrocarbons that have an acid group at one end. The most common fatty acids range between 16 and 22 carbons in length, with some medium-chain fatty acids seen between 12 and 14 carbons long.
The length and the nature of bonds between the carbons in these chains determine their structure. Saturated fatty acids are so-called because all the bonds between the atoms are singular and so no other atoms can be added. Unsaturated fatty acids have the presence of one (monounsaturated) or more than one (polyunsaturated) double bond in the chain.
We typically find fatty acids either by themselves (non-esterified), or bound together with other molecules (esterified). Most of the lipid we find in the diet, and indeed in the body, is in the form of triacyclglyceride (TAG), comprising of three fatty acids joined to a glycerol backbone.
Emulsifying
Given lipids cannot be dissolved in water, this presents a challenge in terms of digestion and absorption. Therefore, lipids are absorbed, and transported, very differently to other macronutrients. Mixing and churning in the stomach helps to emulsify any fat present creating an emulsion of more and smaller suspended in water. This is like other emulsions you may have come across, such as emulsion paint or salad dressing.
As this emulsion leaves the stomach, a specific enzyme, namely pancreatic lipase, breaks down the TAG into fatty acids and glycerol, but these are still not soluble in water and so need to be carried for absorption by the bile salts within bile.
Inside your enterocytes (or intestinal absorptive cells) all the fat, cholesterol and fat-soluble vitamins get packaged inside of protein and phospholipid spheres called chylomicrons so they can be transported in a water solution like blood. These chylomicrons are very large types of lipoproteins (lipid and protein) that serve like shopping bags, allowing for carriage of lipid in the blood.
The chylomicrons drain from your enterocytes into the intestinal lymph and then out into the blood (systemic) circulation, effectively bypassing the liver. This is an important distinction to make, as lipid levels in the blood are not regulated by the liver, or controlled hormonally, in the same way as glucose.
TAG In The Bloodstream
Most of the fat that you eat in your meal simply ends up directly entering the blood as chylomicrons. These very large, TAG filled, shopping bags can then deliver this lipid to tissues for storage or muscle for fuel. In a similar way to how blood glucose rises and falls after eating carbohydrate, your blood TAG levels increase after a fatty meal.
This is so pronounced, that after a fatty meal, your blood can essentially go cloudy with all the fat that is suspended in it. Once these chylomicrons have delivered their contents (mainly TAG), the remnants end up being cleared by the liver, and recycled. Clearance of this fat from the blood typically takes far longer than that of glucose, stretching many hours after the meal itself.
Fat As Energy
From a metabolic perspective, lipids have two main roles, the first relates to their use as energy, and the second relates to their structural and functional role. The use of lipids for energy is probably the most obvious, given that it contains more than double the energy per gram to that of carbohydrate or protein. Having recognised that glucose is the primary fuel used in energy metabolism, lipids can be regarded as being the secondary fuel.
There are some important caveats though. Firstly, the breakdown of fatty acids for energy is slightly laborious compared to glucose and so the rate in which we can liberate energy is relatively slow. This is slightly helped by selecting saturated fatty acids that have no double bonds to deal with when breaking them down.
Another important caveat is that you need to have mitochondria and oxygen present to break down fatty acids, unlike glucose which can be broken down without any of these. The brain cannot use most fatty acids for energy, not because it lacks oxygen supply or mitochondria, but because lipids cannot reach the brain due to the blood brain barrier.
Despite these limitations, it is very handy to have fatty acids as a back-up or supplementary fuel, but you cannot use this as your only universal fuel, especially when considering the metabolic requirement for glucose.
Omega 3 & 6
Beyond energy, lipids also perform an important structural role, as they are the major component of cell membranes. Using de-novo lipogenesis and additional processes in the liver, we can make these longer and more complex (unsaturated) fatty acids, provided we have the right starting materials. Certain unsaturated fatty acids cannot be synthesised by humans due to the location of their double bonds, and so need to be imported from the diet. These are the essential fatty acids.
There are only two truly essential fatty acids, and these are linoleic acid and alpha-linolenic acid, both 18 carbons long. Linoleic acid is an “omega-6” (or n6) fatty acids because it has a double bond 6 carbons in from one end (the omega end), whereas alpha linolenic acid has a double bond 3 carbons in from the end, hence is an omega-3 (n3) fatty acid. From these two essential fatty acids, longer and more complex fatty acids can be synthesised.
The Third Macro: Protein
Protein is the most abundant nitrogen containing compound found in the diet and in our bodies. Proteins are constructed from units of amino acids, all of which contain a nitrogen containing amino group (alkali), alongside a carboxyl group (organic acid). The presence of these two functional groups in all amino acids enables any two or more amino acids to bind to each other.
There may only be around 20 different amino acids that naturally occur in humans, but, which ones are used, in what combination, and how many during protein construction, means that the variability in size, shape, and function of proteins is near infinite. This should not be a surprise when you consider, for example, the number of combinations you could get with a simple four-digit pin code, using just the numbers zero to nine.
The Essential Amino Acids
From the 20 abundant amino acids, we sub-divide these into two main categories, namely “essential” (or non-dispensable) and “non-essential” (or dispensable) amino acids. The essential amino acids, like essential fatty acids, are so called because we cannot synthesise them ourselves in the body, or certainly not at a rate that meets our requirement. Hence we need to import these essential amino acids from the diet.
The current consensus is that there are eight essential amino acids, namely isoleucine, leucine, lysine, methionine, phenylalanine, threonine, tryptophan, and valine. Histidine and arginine are also considered to be essential for infants until such time they can synthesise these themselves after several years of age.
There are also some “semi essential” (or conditionally indispensable) amino acids, which are not themselves essential but rely on a certain supply of other amino acids for their synthesis. Two examples include cysteine which requires methionine, and tyrosine which is made from phenylalanine. There are also some amino acids that may need supplying from the diet at times of greater utilisation, e.g. arginine and glutamine.
Protein Turnover
Within the body there is a continual cycle of protein turnover, with proteins broken down into their amino acid components while at the same time other proteins are constructed from amino acids. Given that protein is needed for growth and repair, plus the obligatory loss of some proteins because of turnover, we need to regularly replenish amino acids (both essential and non-essential) from the diet.
The process of digestion is very involved but ultimately breaks down dietary protein into individual amino acids, which are then actively absorbed (i.e. using energy). Indeed, the whole process of protein assimilation is an energy costly process, equivalent to 20 percent or more of the energy content of the protein itself.
When Protein Stores Are Low
Although we have a lot of protein in our body at any one time, we do not have definitive stores of protein. Muscle represents the largest protein reservoir but is not a functioning store in the traditional sense. We do have a small collective “pool” of amino acids we can use in the blood and tissues, but in order to maintain this pool in times of protein inadequacy, some muscle protein may be compromised to maintain protein synthesis and (other synthesis) elsewhere.
However, excess protein intake will not automatically lead to increased stores in muscle protein, the limited capacity to store new proteins means excess amino acids are typically oxidised into carbon dioxide and ammonium ions (converted to urea for excretion), or converted into other things such as fat or carbohydrate. For these reasons, in healthy adults, under normal circumstances, we maintain a near perfect balance between protein intake and protein loss.
This is an abridged and edited excerpt from Dr. Adam Collins’ upcoming book the Metabolic Manual. To read more of his work click here.